Flanking the double doors leading into Virginia Tech’s Helmet Lab, a history of helmets is mounted to the walls—football on the left, ice hockey on the right. Underneath each helmet—some of which are sawed in half to show how their padding has evolved—is the brand name, the cost, a score and a rating.
The display ushers visitors into the heart of a lab in Blacksburg, Virginia, that since 2007 has been testing sports helmets to determine their ability to reduce concussion risk. For a decade, the lab has been translating its independent research into what it calls a STAR (“Summation of Tests for Analysis of Risk”) rating system for consumers. The ratings—given on a one-to-five scale—indicate how effective a helmet model is in reducing concussion risk for its designed sport. The lab has rated helmets for sports ranging from football to cycling to skiing. Until recently, though, it had not tackled horseback riding helmets.
That changed in 2019, when the lab started crowdfunding to add equestrian helmets to its testing roster, reaching $425,000 in December 2020 through the help of U.S. Equestrian Federation, U.S. Hunter Jumper Association, U.S. Eventing Association and Jacqueline Mars.
As the Helmet Lab prepares to release its equestrian STAR rating system, expected in November, it held an equestrian research symposium last week to share its work. Before that symposium, we had a chance to visit the lab to learn more about the rating system and how it tests helmets with Dr. Barry Miller, director of outreach and business development, and Sydney Moriarty, a rider and research assistant at the lab.
Why Are These Ratings Needed?
Currently, equestrian helmets undergo only a pass/fail certification before they hit the market. That certification, from the American Society for Testing and Materials, indicates whether the helmet will withstand a catastrophic, skull-fracturing impact. ASTM puts each helmet on a wire drop tower, which looks similar to a guillotine, but with a helmet mounted to a head form instead of a blade, which then is dropped onto a steel anvil. If the helmet withstands an impact at a force equivalent to what would cause a skull fracture or death in a real-life situation, it passes.
What ASTM testing does not measure is how the helmets perform at lower impact—like that involved in many riding falls.
“The standard was surprising, learning about it, because it wasn’t necessarily sports specific,” Moriarty said. “It was designed for single impact, high impact, but when we fall, it’s not usually like that.”
Beyond ASTM certification, there has been nothing further for equestrian consumers to distinguish between helmets and determine their relative safety and efficacy. That is what the Helmet Lab’s research is tackling.
“So [ASTM deals with] catastrophic head injury threshold, then we fill the whole void between catastrophic and the rest of all the injuries,” Miller said. “That’s more important than anything else, but we can supplement that with, ‘Alright, now here’s the relative difference between these. They both passed, [but] which one’s better?’ We fill that void with concussion risk, which is our criteria for which one’s better.”
The lab tests how helmets perform against linear and rotational force, the two main ingredients that play into the severity of brain injury when you hit your head.
“The stress and strain of your brain trying to maintain its position inside the skull is what causes the concussion, so it doesn’t have to slam into the side of your skull,” Miller said.
It All Started With Football
Biomedical engineering professors Drs. Stefan Duma and Steve Rowson cofounded the Helmet Lab and released their first set of STAR ratings in 2011. Those ratings were for varsity football helmets, and researchers started by studying the school’s Division 1 Hokies football team. They collected data using sensors placed inside players’ helmets, which helped quantify the probability of each impact leading to a concussion.
“These red dots sit next to your head,” Miller said, showing the sensors in a helmet that was used for collecting data. “So, every time this helmet hits, it’s recorded: every game, every practice, for many, many years. The newer models don’t allow us to put them in, but at this point we have 2 million data points. So, every time that’s happened [it’s recorded]. And if you had a concussion on the field in football, well, that’s paired and diagnosed.”
The data points gave researchers a clear picture of the relationship between linear and rotational acceleration in creating a concussion. The greater the acceleration of one or both, the higher the probability that a person will sustain a concussion from impact.
From this knowledge, researchers were able to create a “combined probability of concussion” graph to help quantify how likely and how severe a concussion could be at certain energy points. That helped them better understand how to measure the effectiveness of a particular helmet at absorbing that energy to reduce acceleration and, in turn, concussion risk.
Helmet manufacturers use different materials and technology from model to model and sport to sport to help absorb energy and reduce acceleration, essentially extending the impact time to reduce concussion risk.
ADVERTISEMENT
To explain that in layman’s terms, Miller uses the example of running into wall: If you ran at full force into a wall, the impact would be short and extremely painful. But if a mattress was padding that wall, it would slow down the moment of impact between you and the wall ever so slightly to literally soften the blow.
That is what helmet manufacturers attempt to do with their padding materials and technology: slow down the moment of impact so the head and the brain inside it, which Miller likens to “thick, wet sand,” hit at reduced linear and rotational force.
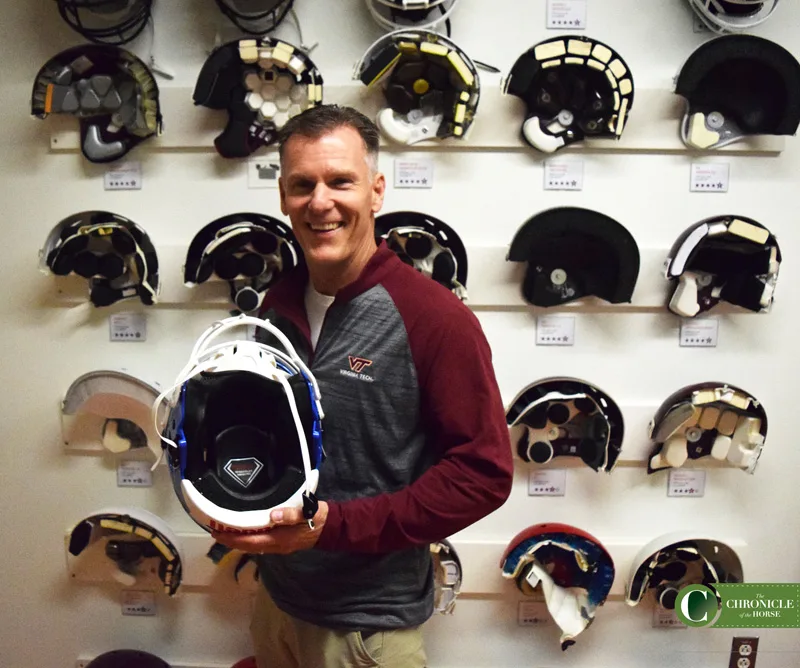
Dr. Barry Miller shows the amount of padding currently found in football helmets to reduce the probability of a concussion.
“[With] these head impacts, we’re talking 5 to 10 milliseconds at the most,” said Miller. “And so if you can buy yourself a little bit more time, spread the curve out, then what happens to that peak? Well, it flattens out. That’s what we want.”
To determine how well helmets work, the researchers created in-lab tests to establish a formula that scores their effectiveness in reducing concussion risk.
“We conduct impact tests in the lab that are representative of real-world scenarios, and the helmets that better reduce head accelerations get better STAR ratings,” said Miller.
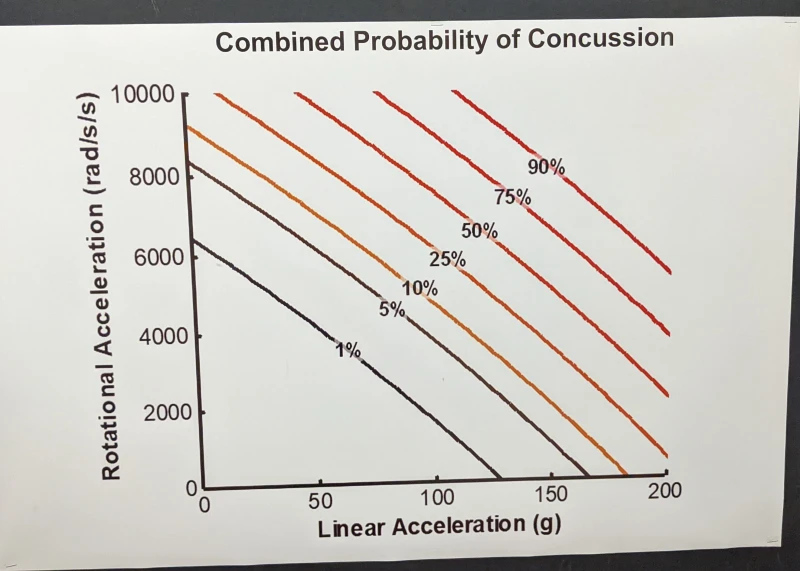
This graph shows the relationship between linear acceleration and rotational acceleration in determining the combined probability of a concussion.
“The STAR score in football represents the average number of concussions you would get wearing that helmet model if you were exposed to the average of 420 seasonal head impacts modeled by our test procedures,” Miller explained.
As the lab expanded to sport beyond football, it would first study how falls happen in each sport—the speeds and force involved in falls and what part of their head participants typically hit, for example—and then develop a unique formula for calculating STAR scores reflective of the variables involved in that sport.
But the “risk function” graph first established for football is a constant in the formula because—whether biking, skiing or riding—the relationship between linear and rotational acceleration doesn’t change.
The best helmets have a low STAR score—meaning a lower average number of concussions expected while wearing that helmet model—which translates to a high STAR rating on the one-to-five scale, to give consumers a better understanding of where a certain helmet sits, comparatively, amongst others on the market.
Going through various sporting helmets in Blacksburg, Miller pulls out brands that have answered this call by doing things such as adding padding, putting in pressure plates that move independently from the rest of the helmet, and creating two layers within the helmet to decouple its movement from that of the head.
(Fun fact: In the riding helmet debate about whether wearing long hair inside or outside the helmet is safer, Miller said hair up can be helpful:
“The retention system—whatever it is for that given helmet—as long as it keeps the helmet in place for that initial impact, it’s done its job,” he said. “If the helmet moves after or during that initial impact—you hit, and it slides down the side of your head, that’s OK; that’s better than it being so coupled that it moves and it takes your head with it. So, hair is usually a good thing. It adds more to the slip plane.”)
Making It Equestrian Specific
“I think there’s lots of improvement opportunities for equestrian helmets,” Miller said. “I don’t think they’ll get much bigger, but I think just incorporating some of that padding material, structures and technology into those helmets.”
For example, traditional, built-in visors should be rethought, Miller said.
“They need to be click-in, click-out or just breakable,” he said. “If they’re molded into the helmet, that’s usually bad because that’s like an edge that can catch.”
When the Helmet Lab began studying riding helmets, its scientists started with background research, which involved reading equestrian literature and watching hundreds of videos of riding falls.
“Stefan did all this video analysis,” Miller said. “So, when a person falls from the horse, their head is usually around the shoulder height of the horse and then it’s a free fall. So, what’s the acceleration from there to the ground? We calculate all that.”
ADVERTISEMENT
In addition, during this background stage, researchers found that in most horse falls, the rider fell nearly vertically from that shoulder height, and that the horse’s forward trajectory didn’t present a major factor.
“[Duma] concluded that out of a hundred videos—which is a lot to actually watch, fully watch and diagnose and break down—almost all the falls are near vertical, by the time you brace and you’re falling off. So, there’s not a lot of horizontal component to them.”
The researchers also conducted field experiments by taking a portable pendulum test rig and a device that measures surface hardness to a nearby equestrian facility. By dropping a dummy head on sand footing, harder high-clay-content footing, grass and artificial footing, they measured head accelerations and established the boundary conditions, which they then applied in the lab to create a fair, standardized testing.
With this field work and data of the “pulses”—the acceleration curve measured during each crash test—they moved back into the lab to create a test rig to mimic real-life situations.
“We dropped helmets—we did helmets on sand; we did helmets on hard dirt. So, we know what those pulses are,” said Miller. “And so, we’re going to try to best match those with one of the test rigs or even build a test rig.”
In all, the Helmet Lab evaluated 26 helmet models and 104 helmets in 312 tests.
To best do this, they decided to use an already established pendulum test rig for mimic equestrian impacts. This pendulum was also used for football, hockey and rugby. In the final phase of their research, the Helmet Lab team is analyzing and evaluating the data collected to create a STAR formula unique to riding and establish their STAR ratings for riding helmets.
One thing unusual about horseback riding is that it encompasses many different situations—and potentially different needs in a helmet, Moriarty noted.
For example, certain padding materials are better for certain types of situations. One type of expanded foam used in some riding helmets is made to crush, crumble and break upon impact. However, Helmet Lab researchers found while such technology works in high-energy impacts, it didn’t perform in low-energy situations.
“What if the energy threshold does not crush, crumble or break the helmet? Then what happens?” Miller said. “Does it attenuate any energy? No. So then it all gets transferred to your head.
“If you’re on a trail ride, walking, and your horse bucks you off, that’s not probably not going to be high impact for it to break,” Moriarty added. “But if you get kicked in the head, on cross-country while you’re galloping, with a stud in the shoe? That might qualify.”
Because of this, equestrian helmets may require different certification standards to account for different uses or disciplines.
Helping Consumers
Once finalized, the STAR rating system will allow consumers to compare equestrian helmets based on their performance and provide more information about their ability to reduce concussion risk. Ideally, the Helmet Lab’s research also will help manufacturers by providing information that allows them to improve helmet design.
The prospect is appealing to officials from organizations, including USEF and USHJA, who helped fund the equestrian-specific research.
“I get dozens of people and parents asking me which helmet is the safest,” Joe Dotoli, USHJA board member and Safety Committee chair, said in a statement from USHJA. “This is the next big step. This tells us the ones that perform better. There are no rule changes here; this is information only. This is being able to go on to the Virginia Tech website, look at the rating and see how your helmet performed for linear and rotational force. The stars are not random; they translate to numbers. The higher the number in the star rating, the better the helmet.
“The result,” he added, “is we are going to have a lot more information so we can make better-informed purchases, and helmet makers can make better helmets.”
To learn more about Virginia Tech Helmet Lab’s helmet rating system, click here.